4.6 Prediction of snow vs rain
LEARNING OBJECTIVES:
|
Evaluate the surface and upper-level synoptic situations in
determining the form of precipitation in your forecast.
|
Typically, an inch or so of precipitation in the form of rain will cause no
serious inconvenience. On the other hand the same amount of precipitation in the
form of snow, sleet, or freezing rain can seriously interfere with naval
operations. In such cases, the snow versus rain problem may become
a factor of operational significance.
Sleet and freezing rain, which often may occur in the intermediate period
between snow and rain, are generally grouped with snow in our discussion. Any
decision arrived at for the snow versus rain problem would, naturally, have to
be modified, dependant on your geographical location. This should be easily
accomplished through a local study of the optimum conditions. The various
techniques and systems presented here will often complement each other. The
approach used here is a discussion of the general synoptic patterns and the
thermal relationship; that is, the use of temperatures at the surface and aloft,
and the presentation of an objective technique to distinguish the types of
precipitation.
The forecasting problem of snow versus rain arises, naturally, during the
colder months of the year. In midwinter when the problem is most serious in the
northern states, the southern states may not be concerned.
The type of precipitation that reaches the ground in a borderline situation
is essentially dependent on two conditions.
There must be a stratum of above-freezing temperatures between the ground and
the level at which precipitation is forming, and this stratum must be
sufficiently deep to melt all of the falling snow prior to striking the surface.
Thus, a correct prediction of rain or snow at a given location depends largely
on the accuracy with which the vertical distribution of the temperature,
especially the height of the freezing level, can be predicted. On the average,
it is generally satisfactory to assume that the
freezing level must be at least 1,200 feet above the surface to ensure that most
of the snow will melt before reaching the surface.
In the lower troposphere, above the surface, horizontal advection is usually
the dominant factor affecting local temperature changes. In most precipitation
situations, particularly in borderline situations, warm air advection and upward
motion are occurring simultaneously, giving rise to the fact that warming
generally accompanies precipitation. However, this effect is frequently offset
when there is weak warm advection, or even cold advection, in the cold air mass
in the lower layers.
In situations where precipitation is occurring in association with a cold upper
low, upward motion is accompanied by little, if any, warm advection. In such
borderline cases, precipitation may persist as snow, or tend to turn to snow,
due to cooling, as a result of upward motion or advection.
The most important of the
nonadiabatic effects taking place during the precipitation process is the
cooling, which takes place due to evaporation as the precipitation falls
through unsaturated air between the clouds and the surface. This effect is
especially pronounced when very dry air is present in the lower levels, with
wet-bulb temperatures at or below freezing.
Then, even if the dry-bulb temperature is above freezing in a layer deeper than
1,200 feet in the lower levels, the precipitation may still fall as snow, since
the evaporation of the snow will lower the temperatures in the layer between the
cloud and the surface until the below-freezing wet-bulb temperatures are
approached. The actual cooling that occurs during the period when evaporation is
taking place may often be on the order of 5° to 10°F within an hour. After
the low-level stratum becomes saturated, evaporation practically ceases, and
advection brings a rise in temperature in the low levels. However,
reheating often comes too late to bring a quick change to rain since the
temperatures may have dropped several degrees below freezing, and much snow may
have already fallen. The lower levels may be kept cool through the transfer of
any horizontally transported heat to the colder, snow-covered surface.
Melting snow descending through
layers that are above freezing is another process which cools a layer. To
obtain substantial temperature changes due to melting, it is necessary to have
heavy amounts of precipitation falling, and very little warm air advection. As
cooling proceeds, the temperature of the entire stratum will reach freezing, so
that a heavy rainstorm could transform into a heavy snowstorm, Incidents of
substantial lowering of the freezing level due to melting are relatively rare.
The combination of heavy rain, and little, if any, warm advection is an
infrequent occurrence.
The combined effects of horizontal temperature advection, vertical motion,
and cooling due to evaporation are well summarized by observations of the
behavior of the bright band on radar (approximately 3,000 ft). Observers have
found that within the first 1 1/2 hours after the onset of precipitation, the
bright band lowers by about 500 to 1,000 feet. This is attributable primarily to
evaporational cooling, and probably secondary to melting. Since evaporational
cooling ceases as saturation is reached, warm air advection, partially offset by
upward motion, again becomes dominant, and the bright band ascends to near its
original level. The bright band will ascend to its original level approximately
3 hours after the onset of precipitation, and may ascend a few thousand
additional feet.
Other nonadiabatic effects, such as radiation and heat exchange with the
surface, probably play a relatively smaller role in the snow-rain problem.
However, it is likely that the state of the underlying surface (snow-covered
land versus open water) may determine whether the lower layers would be above or
below freezing. Occasionally, along a seacoast in winter, heat from the open
water keeps temperatures offshore above freezing in the lower levels. Along the
east coast of the United States, for example, coastal areas may have rain, while
a few miles inland snow predominates. This situation is associated with
low-level onshore flow, which is typical of the flow associated with many east
coast cyclones. Actually, this situation cannot be classified as a purely
nonadiabatic effect since the warmer ocean air is being advected on shore.
The snow versus rain problem usually depends upon relatively small-scale
synoptic considerations, such as the exact track of the surface disturbance,
whether the wind at a coastal station has an onshore component, the position of
the warm front, and the orientation of a ridge east of the low.
In the larger sense, the snow versus rain zone is directly related to the
position of the polar front. The location of the polar front is, in turn,
closely related to the position of the belt of strong winds in the middle and
upper troposphere. When the westerlies extend farther to the south, storm tracks
are similarly affected, and the snow-rain zone may be farther to the south. As
the westerlies shift northward of their normal position, the storm tracks
develop across Canada. Concurrent with this northward shift, the United States
has above normal temperatures, and the snow-rain problem exists farther to the
north.
With a high zonal index situation
aloft, the snow-rain zone will extend in a narrower belt, often well ahead of
the surface perturbation and will undergo little north-south displacement, Those
areas with precipitation occurring will not undergo a change from one form to
another since there is relatively little advection of warm or cold air with a
high zonal condition.
When the upper-level wave is of large or increasing amplitude (low
zonal index), it is difficult to generalize about the characteristics of
the snow versus rain problem without considering the surface perturbation. Up to
this point, we have discussed the snow-rain pattern in association with an
active low of the classical type, The rate of precipitation accumulation here is
rapid, and the transition period of freezing rain or sleet is short, usually on
the order of a few hours or less. Another situation in which there is frequently
a snow versus rain problem is that of a quasi-stationary
front in the southern states, with a, broad west-southwest to southwest
flow aloft, and a weak surface low. The precipitation area in this case tends to
become elongated in the direction of the upper-level current. The precipitation
rate may be slow, but it occurs over a longer period. Often a broad area of
sleet and freezing rain exists between belts of snow and rain, leading to a
serious icing condition over an extensive region for a period of several hours
or more. This pattern of precipitation changes either as an upper trough
approaches from the west and initiates cyclogenesis on the front or as the flow
aloft veers and precipitation ceases.
Approaches to the snow versus rain forecasting problem have generally fallen
into three broad categories.
The first category depends on the use of observed flow patterns and
parameters to predict the prevalent form of precipitation for periods as much as
36 hours in advance.
The second category consists of studies relating local parameters to the
occurrence of rain or snow at a particular station, or area. In this approach,
it is assumed thermal parameters will be obtainable from prognoses. This
approach tends to have its greatest accuracy for periods of 12 hours, or less,
since longer periods of temperature predictions for the boundary zone between
rain and snow are very difficult to make with sufficient precision.
A third category used involves the use of one of the many objective
techniques available. A number of stations have developed objective local
techniques. The method presented here is applicable to the eastern half of the
United States. Thus, the general procedure in making a snow versus rain forecast
at present is to use a synoptic method for periods up to 24 or 36 hours, and
then consider the expected behavior of thermal parameters over the area to
obtain more precision for periods of about 12 hours or less.
A number of methods based on synoptic flow patterns applicable to the United
States are described in the U.S. Department of Commerce's publication, "The
Prediction of Snow vs Rain", Forecasting Guide No. 2. These methods are
mostly local in application and are beyond the scope of this manual.
Prognostic charts from the National Meteorological Center and other
sources should be used whenever and wherever available, not only to determine
the occurrence and extent of precipitation, but for the prediction of the
applicable thermal parameters as well.
The following text discusses methods of employing surface temperature,
upper-level temperatures, 1000- to 700-hPa and 1000- to 500-hPa thicknesses, the
height of the freezing level, and combined parameters for the prediction of snow
versus rain. All of these are interdependent, and should be considered
simultaneously.
SURFACE TEMPERATURE.- Surface temperature considered by itself is not an
effective criterion. Its use in the snow versus rain problem has generally been
used in combination with other thermal parameters. One study for the
Northeastern United States found that at 35°F snow and rain occurred with equal
frequency, and by using 35°F as the critical value (predict snow at 35°F and
below, rain above 35°F), 85 percent of the original cases could be classified,
Another study based on data from stations in England suggested a critical
temperature of 34.2°F, and found that snow rarely occurs at temperatures higher
than 39°F. However, it is obvious from these studies that even though surface
temperature is of some value in predicting snow versus rain, it is often
inadequate. Thus, most forecasters look to upper-level temperatures as a further
aid to the problem.
UPPER-LEVEL TEMPERATURES.- Two studies of the Northeastern United States
found that temperatures at the 850-hPa
level proved to be a good discriminating parameter, and that including
the surface temperature did not make any significant contribution. The
discriminating temperatures at the 850-hPa level were -2° to -4°C. Another
study found that the area bounded by the 0°C isotherm at 850-hPa level and the
32°F isotherm at the surface, when superimposed upon the precipitation area,
separated the snow-rain precipitation shield in a high percentages of cases. A
range of -2° to -4°C at the 850-hPa level should be used along coastal areas,
and also behind deep cold lows, At mountain stations a higher level would have
to be used.
A technique that uses temperatures at mandatory levels (surface, 1000-,
850-, 700-, and 500-hPa, etc.) is advantageous because of the availability of
charts at these levels. There is, however, the occasional problem where
temperature inversions are located near the 850- or 700-hPa levels, so that the
temperature of one level may not be indicative of the layer above or below. This
difficulty can be overcome by using thickness, which is a measure of the mean
temperature of the layer.
THICKNESS.- The National Weather Service has examined both the 1,000- to
700-hPa and 1,000- to 500-hPa thickness limits for the eastern half of the
United States.
A generalized study of 1,000- to 500-hPa thickness as a predictor of
precipitation forms in the United States was made by A. J. Wagner. More complete
details on this study can be found in The Prediction of Snow vs Rain,
Forecasting Guide No.2.
Wagner's data was taken from a study of 40 locations in the United States for
the colder months of a 2-year period. Cases were limited to surface temperatures
between 10°F and 50°F. The form of precipitation in each case was considered
as belonging in one of two categories-frozen which includes snow, sleet,
granular snow, and snow crystals; and unfrozen, which includes rain, rain and
snow mixed, drizzle, and freezing rain and drizzle.
Equal probability, or critical thickness values, were obtained from the data at
each location. From this study it was clear that the
critical thickness values increase with increasing altitude. This
altitude relationship is attributable to the fact that a sizable portion of the
thickness stratum is nonexistent for high-altitude stations, and obviously does
not participate in the melting process. To compensate for this, the equal
probability thickness values must increase with station elevation. For higher
altitude stations, thickness values between the 850- to 500-hPa or 700- to
500-hPa stratums, as appropriate, should prove to be better dated to
precipitation form.
The Wagner equal probability chart is reproduced in figure 4-21.
Wagner's study also indicates that the form of precipitation can be specified
with a certainty of 75 percent at plus or minus 30 meters from the equal
probability value, increasing to 90 percent certainty at plus or minus 90 meters
from this value.
Stability is the parameter that accounts for the variability of precipitation
for a given thickness at a given point. This fact is taken into account in the
following reamer: if the forecast
precipitation is due to a warm front that is more stable than usual, the line
separating rain from frozen precipitation is shifted toward higher thickness
values. Over the Great Lakes, where snow occurs in unstable, or stable
conditions, the equal probability thickness is lower than that shown in figure
4-21 for snow showers, and higher than that shown in figure 4-21 for warm
frontal snow.
HEIGHT OF THE FREEZING LEVEL.- The
height of the freezing level is one of the most critical thermal parameters in
determining whether snow can reach the ground. It was pointed out earlier
that theoretical and observational evidence indicates that a freezing level
averaging 1,200 feet or more above the surface is usually required to ensure
that most of the snow will melt before reaching the surface. This figure of
1,200 feet can thus be considered as a critical or equal probability value of
the freezing level.
COMBINED THERMAL PARAMETERS.- From the foregoing discussion, you can
conclude that no one method, when used alone, is a good discriminator in the
snow versus rain forecasting problem. Therefore, you should use a combination of
the surface temperature, height of the freezing level, 850-hPa temperature, and
the 1,000-to 700-hPa and /or 1,000- to 500-hPa thicknesses to arrive at the
forecast, There is generally a high correlation between the 850-hPa temperature,
and the 1,000- to 700-hPa thickness and between the 700-hPa temperature and the
1,000- to 500-hPa thickness. Certainly an accurate temperature forecast for
these two levels would yield an approximate thickness value for discriminating
purposes.
The determining factor in the form of precipitation in this study was found
to be the distribution of temperature and moisture between the surface and the
700-hPa level at the time of the beginning of precipitation. The median level of
850-hPa was studied in conjunction with the precipitation area and the 32°F
isotherm sketched on the surface chart. This method presents an objective and
practical method by which the forecaster can make a decision on whether the
precipitation in winter will be rain, snow, freezing rain, sleet, or some
combination of these.
Figure 4-21.-Map showing 1,000- to 500-hPa thickness values
for which probability of rain or frozen precipitation is equal (after
Wagner).
|
The following objective techniques can be applied to the land areas south of
50° north latitude and east of a line drawn through Williston, North Dakota;
Rapid City, South Dakota; Goodland, Kansas; and Amarillo, Texas.
The area outlined by the 0°C isotherm at 850-hPa and the 32°F isotherm on the
surface chart, when superimposed upon the precipitation area, generally
separates the forms of precipitation. Most of the pure rain was found on the
warm side of the 32°F isotherm, and most of the pure snow on the cold side of
0°C isotherm, with intermediate types falling generally within the enclosed
area between these two isotherms. It was also observed that in a large majority
of situations, evaporation and condensation was a sizable factor, both at
850-hPa and at the surface level in its affect upon temperature.
With this in mind, the wet-bulb
temperature was selected for investigation because of its conservative
properties with respect to evaporation and condensation, and also because of its
ease of computation directly from the temperature and dewpoint. The surface
chart is used for computations of the 1,000-hPa level because the surface chart
approximates the 1,000-hPa level for most stations during a snow situation;
therefore, little error is introduced. IT MUST BE REMEMBERED THAT ALL
PREDICTIONS ARE BASED ON FORECAST VALUES.
MOVEMENT OF THE 850-hPa 0°C ISOTHERM.- A reasonably good approximation for
forecasting the 0°C isotherm at the 850-hPa level can be made subjectively by
use of a combination of extrapolation and advection, considerations of synoptic
developments, and the rules listed in the following paragraphs. (See figure
4-22, views (A) and (B), for typical warm and cold air advection patterns at
850-hPa.)
The following rules for the movement of the 24-hour, 850-hPa level temperature
change areas have been devised
-
Maximum cooling takes place between the 850-hPa contour trough and the
850-hPa isotherm ridge east of the trough.
-
Maximum warming takes place between the 850-hPa contour ridge and the
850-hPa isotherm trough east of the contour ridge.
-
Changes are slight with ill-defined isotherm/contour patterns.
-
Usually, little change occurs when isotherms and contours are in phase at
the 850-hPa level.
-
The temperature falls at the 850-hPa level tend to replace height falls at
the 700-hPa level in an average of 24 hours. Conversely, temperature rises
replace height rises.
-
With filling troughs or northeastward moving lows, despite northwest flow
behind the trough, 850-hPa level isotherms are seldom displaced southward, but follow the trough toward the
east or northeast . Always predict temperature falls immediately following a
trough passage.
-
Do not forecast temperature rises of more than 10 to 2°F in areas of light or
sparse precipitation in the forward areas of the trough. If the area of
precipitation is widespread and moderate or heavy, forecast no temperature rise.
-
With eastward moving systems under normal winter conditions (trough at the
700-hPa level moving east about 10° per day), a distance of 400 nautical miles
to the west is a good point to locate the temperature to be expected at the
forecasting point 24 hours later. A good 850-hPa temperature advection speed
seems to be about 75 percent of the 700-hPa trough displacement.
|
Figure 4-22.-Typical cold and warm air advection patterns at 850-hPa. (A)
Cold; (B) Warm.
|
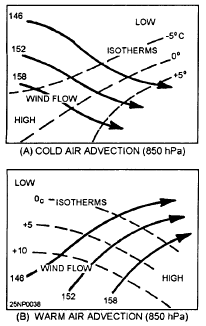 |
The following is step-by-step procedures for moving the 850-hPa 0°C isotherm:
-
Extrapolate the movement of the thermal ridge and troughs for 12 and 24 hours.
If poorly defined, this step may be omitted. The amplitude of the thermal wave
may be increased or decreased subjectively if, during the past 12 hours, there
has been a corresponding increase or decrease in the height of the contours at
500-hPa.
-
The thermal wave patterns will maintain their approximate relative position
with the 850-hPa level contour troughs and ridges. Therefore, the 12- and
24-hour prognostic positions of the contour troughs and ridges should be made,
and the extrapolated positions of the thermal points checked against this
contour prognosis. Adjustments of these thermal points should be made.
-
Select points on the 0°C isotherm that lie between the thermal ridge and
trough as follows: one or two points in the apparent warm advection area, and
one or two points in the apparent cold advection area. Apply the following rules
to these selected points.
-
Warm advection area. If the points lie in a near saturated or precipitation
area, they will remain practically stationary with respect to the contour trough.
If the points lie in a nonsaturated area, but one that is expected to become
saturated or to lie in precipitation area, then it will remain stationary or
move upwind slightly to approximate y the prognostic position of the 0°C
wet-bulb. If the point does not fall in the above two categories, it will be
advected with about 50 percent of the wind component normal to the isotherm.
Note in all three cases above, the movement is related to the contour pattern.
-
Cold Advection area. Advect the point with approximately 75 to 80 percent of
the wind component normal to it.
In the case of a slow moving, closed low at the 850-hPa level, the 0°C isotherm
will move eastward with respect to the closed low as cold air is advected around
the low.
WET-BULB ISOTHERM.- The wet-bulb temperature can be forecast by the above
procedures and rules. Remember that the wet-bulb temperature is dependent upon
dewpoint as well as the temperature. The dewpoint will be advected with the
winds at nearly the full velocity, whereas the temperature under nonsaturated
conditions moves slower. The following observations with respect to the 0°C
wet-bulb isotherm after saturation is reached may help:
-
The 0°C wet-bulb isotherm does not move far offshore in the Gulf and the
Atlantic because of upward vertical motion in the cold air over the warmer water.
-
If the 0°C wet-bulb isotherm lies in a ribbon of closely packed isotherms,
movement is slow.
-
Extrapolation works well on troughs and ridges. After the forecast of the
surface and 850-hPa level temperature and dewpoint values are made, you are
ready to convert these values to their respective wet-bulb temperature. The
following procedures are recommended
-
Use figure 4-23, views (A) and (B), to compute the wet-bulb temperatures for
the 1,000- and 850-hPa levels, respectively. (The surface chart may be used for
the 1,000-hPa level.) Admittedly, the wet-bulb temperatures at just these two
levels do not give a complete picture of the actual distribution of moisture and
temperature, and error is introduced when values are changing rapidly, but these
are values the forecaster can work with and predict with reasonable accuracy.
-
Refer to figure 4-24. From the surface wet-bulb temperature at the bottom, go
up vertically until you intersect the computed 850-hPa level wet-bulb
temperature to the left. This intersection indicates the form of precipitation
that can be expected. A necessary assumption for use of this graph is that the
wet-bulb temperatures at these two levels can be predicted with reasonable
accuracy. Known factors affecting the wet-bulb temperature at any particular
station should be carefully considered before entering the graph.
Some of the known factors are elevation, proximity to warm bodies of water,
known layers of warm air above or below the 850-hPa level, etc. Area
"A" on the graph calls for a rain forecast, area "B" for a
freezing rain forecast, and area "C" for a snow forecast. Area
"D" is not so clear cut because it is an overlap portion of the graph;
however, wet snow or rain and snow mixed predominate in this area. Sleet
occurring by itself for more than 1 or 2 hours is rare, and should be forecast
with caution.
The intent of this section is to introduce the patterns associated with maximum
snowfall and to present techniques for predicting the areas where snowstorms are
likely to appear.
There are four distinct types of synoptic patterns with associated maximum snow
area.
BLIZZARD TYPE.- The synoptic situation features an occluding low. In the
majority of cases, the "wrapped around" high pressure and ridges are
present. The track of the low is north of 40°N, and its speed, which initially
may be average or about 25 knots, decreases into the slow category during the
occluding process. In practically all cases, a cold closed low at the 500-hPa
level is present and captures the surface low in 24 to 36 hours.
The area of maximum snowfall lies to the left of the track. At any particular
position, the area is located from due north to west of the low-pressure center.
When this type occurs on the east coast with its large temperature contrast and
high moisture availability, heavy snowfall may occur. The western edge of the
maximum area is limited by the 700-hPa level trough, or low center, and the end
of all snow occurs with the passage of the 500-hPa level trough or low center.
MAJOR STORM AND NONOCCLUDING LOWS.- The synoptic situation consists of a
nonoccluding wave-type low. The track of the low or wave is south of 40°
latitude, and its speed is at least the average of 25 knots, often falling into
the fast-moving category. The upper-air picture is one of fast-moving troughs,
generally open, but on occasion could have a minor closed center for one or two maps in the bottom of the trough. The area of
maximum snowfaIl lies in the cold air to the left of the track and usually
describes a narrow belt oriented east-west or northeast-southwest about 100 to
200 miles wide. At any position, the area is parallel to the warm front and
north of the low-pressure center. Within the maximum area, the rate of snowfall
is variable from one case to another, depending upon available moisture, amount
of vertical shear, etc. However, it is not uncommon for heavy snow (1 inch per hour or greater) to occur. You must remember that even though heavy snow
occurs, the duration is short, This means that a location could lie in the
maximum snow area only 4 to 8 hours; whereas, in the case of the blizzard type,
it usually remains in the area in excess of 10 hours.
. |
4-23.-Graphs for computing wet-bulb temperatures. (A) Computation of
1,000-hPa level wet-bulb temperature; (B) computation of 850-hPa level wet-buib
temperature
|
Figure |
WARM ADVECTION TYPE.- This type was separated from the other types because of
the absence of an active low in the vicinity of the maximum snow area. A
blocking high or ridge is located ahead of a sharp warm front. The overrunning
warm air is a steady current from the south to southwest. The area of maximum
snowfall is a narrow band parallel to the warm front and moves north or
northeast. A rate of fall of moderate to heavy for a 6- to 12-hour duration may
occur. The usual history is a transition to freezing rain, and then rain.
POST-COLD FRONTAL TYPE.- The synoptic situation consists of a sharp cold
front oriented nearly north-south in a deep trough. A minor wave may form on the
front and rapidly travel to the north or northeast, Strong cold advection from
the surface to the 850-hPa level is present west of the front. The troughs at
the 700-hPa and 500-hPa levels are sharp and displaced to the west of the
surface trough 200 to 300 miles. Ample moisture is available at the 850-hPa and
700-hPa levels. This type of heavy snow may occur once or twice per season.
The area of maximum snowfall is located between the 85MPa and 700-hPa troughs,
where moisture at both levels is available, The rate of fall is moderate,
although for a brief period of an hour or less, it may be heavy. The duration is
short, on the order of 2 to 4 hours at anyone location. The area as a whole
generates and dissipates in a 12- to 18-hour period.
The normal history is one of a general area of light snow within the first 200
miles of a strong outbreak of cold air. After the cold air moves far enough
south and the cold front becomes oriented more north to south and begins moving
steadily eastward, the troughs aloft and moisture distribution reach an ideal
state, and a maximum snow area appears. After 12 to 18 hours, the advection of
dry air at the 700-hPa level decreases the rate or fall in the area, and soon
thereafter, the area, as a whole, dissipates.
4.6.6.2 Locating the Area of Maximum Snowfall
The various parameters and characteristics that may be of benefit in locating
areas of maximum snowfall are discussed in he following text.
TEMPERATURE.- The 0°C (-3°C east coast) isotherm at the 850-hPa level is used
as a basis for snow-rain areas, This sotherm should be carefully analyzed by
using all data at 850 hpa. It should then be checked against the surface chart.
Keep in mind the following two points:
In areas of precipitation, locations reporting snow should lie on the cold
side of the 0°C (-3°C east coast) isotherm; for ocations reporting mixed types
of precipitation (e.g., rain and snow, sleet and snow), the 0°C (-3°C)
isotherm will lie ery close to or through the location.
In areas of no precipitation, the 0°C (-3°C east coast) isotherm will
roughly parallel the 32°F isotherm at the surface. In cloudy areas, the
separation will be small, and in clear areas, the separation will be larger. At
the 850-hPa level, the 0°C wet-bulb temperature should be sketched in,
particularly in the area where precipitation may be anticipated within the next
12 to 24 hours. This line will serve as the first approximation of the future
position of the 0°C isotherm.
MOISTURE.- At the 850-hPa level, the -5°C dewpoint line is used as the basic
defining line; at the 700-hPa level, the -10°C dewpoint line is used as the
basic defining line. The area at 850 hpa that lies within the overlap of the
0°C isotherm and the -5°C dewpoint line is the first approximation of the
maximum snowfall area. All locations within this area have temperatures less
than 0°C and spreads of 5°C or less. This area is further refined by
superimposing the sketched -10° dewpoint line at 700 hPa upon the area. Now the
final area is defined by the 0°C isotherm and the overlapped minimum dewpoint
lines from both levels. This final area becomes the area where moderate or heavy
snow will be reported, depending upon the particular synoptic situation. See figure 4-25,
MOVEMENT.- The first basic rule for moving the area of maximum snowfall is that
it maintains the same relative position to the other synoptic features of the
850-hPa level and surface charts. However, in order to forecast the expansion or
contraction of the area, it is necessary to forecast the lines that define it.
The 0°C isotherm should be forecast according to the roles set forth in the
section treating this particular phase. The moisture lines may be advected with
the winds. The 0°C isotherm should also be moved with rules stated previously
in this chapter.
The area of maximum snowfall can be forecast for 12 hours with considerable
accuracy, and for 24 hours with fair accuracy, provided a reasonable amount of
care is exercised according to rules and subjective ideas mentioned previously.
Figure 4-25.-Illustration of the location of the maximum snow area. The low
center moved to Iowa in 24 hours, and the maximum snow area spread northeast
along the area 50 to 75 miles either side of a line through Minneapolis to
Houghton, Michigan.
|
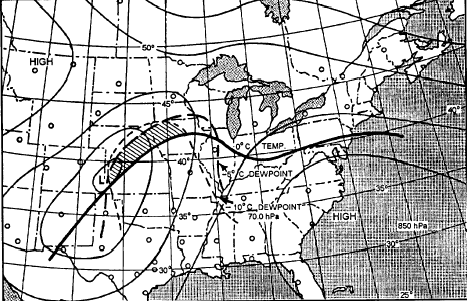 |
|