4.5 Cloud analysis and forecasting
LEARNING OBJECTIVES:
|
Recognize upper air data and its value in forecasting. Recognize
moisture features aloft and their significance to the forecaster.
|
Forecasters are frequently called
upon to make forecasts of clouds over areas where synoptic observations are not
readily available, or over areas where clouds above the lowest layer are
obscured by a lower cloud deck.
This section is designed to acquaint the forecaster with the principles of
detection and analysis of clouds from rawinsonde data. A complete discussion of
this problem is beyond the scope of this training manual. Further information on
this subject may be found in the practical training publication, "Use of
the Skew T Log P Diagram in Analysis and Forecasting", NAVAIR 50-1P-5.
Cloud observations regularly available to forecasters in surface synoptic
reports leave much to be desired as a basis for cloud forecasting.
Rawinsondes, which penetrate cloud systems, reflect, to some extent (primarly in
the humidity trace), the vertical distribution of clouds. If the humidity
element were perfect, there would usually be no difficulties in locating cloud
layers penetrated by the instrument. Because of the shortcomings in the
instrument, however, the relationship between indicated humidity and cloud
presence is far from definite, and art empirical interpretation is necessary.
Nevertheless, rawinsonde reports give valuable evidence that, when compared with
other data, aids greatly in determining a coherent picture of stratiform and
frontal cloud distributions. Their value in judging air mass cumulus and
cumulonimbus distribution is negligible.
Theoretically, we should be able to infer from the humidity data of RAOBs
the layers where the rawinsonde penetrates cloud layers. In practice, the
determination that can be made from temperature and dewpoint curves are often
less exact and less reliable than desired. Nevertheless, RAOBs give clues about
cloud distribution and potential areas of cloud formation. These clues generally
cannot be obtained from any other source.
The temperature minus the dewpoint depression yields the dewpoint,
which is defined as the temperature to which the air must be cooled at a
constant vapor pressure for saturation to occur. The FROST POINT (that is, the
temperature to which the air has to be cooled or heated adiabatically to reach
saturation with respect to ice) is higher than the dewpoint except at 0°C,
where the two coincide. In the graph shown in figure 4-15, the difference
between dewpoint and frost point is plotted as a function of the dewpoint itself.
Figure 4-15.-
Difference between frost point and dewpoint as
a function of the dewpoint.
|
In a cloud with the temperature above freezing, the true dewpoint will
coincide closely with the true air temperature, indicating that the air between
the cloud droplets is practically saturated. Minor discrepancies may occur when
the cloud is not in a state of equilibrium (when the cloud is dissolving or
forming rapidly, or when precipitation is falling through the cloud with
raindrops of slightly different temperature than the air); but these
discrepancies are very small. In the subfreezing portion of a cloud, the true
temperature is between the true dewpoint and the true frost point, depending on
the ratio between the quantities of frozen and liquid cloud particles. If the
cloud consists entirely of supercooled
water droplets, the true temperature and the true dewpoint will, more or
less, coincide. If the cloud consists entirely of ice,
the temperature should coincide with the frost point. Therefore, we cannot look
for the coincidence of dewpoint and temperatures as a criterion for clouds at
subfreezing temperatures. At temperatures below -12°C, the temperature is more
likely to coincide with the frost point than the dewpoint. The graph shown in
figure 4-15 indicates that the difference between the dewpoint and frost point
increases roughly 1°C for every 10°C that the dewpoint is below freezing.
For example, when the dewpoint is -10°C, the frost point equals -9°C; when
the dewpoint is -20°C, the frost point is -18°C; and when the dewpoint is
-30°C, the frost point is
-27°C. Thus, for a cirrus cloud that is in equilibrium (saturated with respect
to ice) at a (frost point) temperature of -40°C, the correct dewpoint would be
-44°C, (to the nearest whole degree).
We can state, in general, that air in a cloud at temperatures below about -12°C
is saturated with respect to ice, and that as the temperature of the cloud
decreases (with height), the true frost point/dewpoint difference increases. Any
attempt to determine the height of cloud layers from humidity data of a RAOB is,
there fore, subject to error. It is possible to overcome some of these errors by
a subjective interpretation of the Rawinsonde Observations (RAOBs), as discussed
in the following sections.
The following diagrams (figs, 4-16, 4-17, and 4-18) illustrate the behavior
of a rawinsonde during cloud penetration.
These diagrams are correlated with aircraft observations or the heights of cloud
bases and tops from aircraft flying in the vicinity of an ascending rawinsonde.
The difference in time and distance between the aircraft and sounding
observations was usually less than 2 hours and 30 miles, respectively. Some of
the aircraft reported only the cloud observed above 15,000 feet; others reported
all clouds. In figure 4-16 through 4-18, the aircraft cloud observations are
entered in the lower left corner of each diagram under the heading cloud; the
surface weather report is entered under the aircraft cloud report.
|
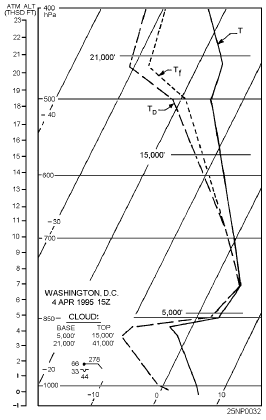 |
|
Figure 4-16.-Example of inferring clouds from a RAOB with an
active warm front approaching from the south.
|
Where the low cloud was not reported by the aircraft, the height of the
cloud base may be obtained from the surface reports, Aircraft height reports are
expressed in thousands of feet, pressure-altitude.
The temperature, frost point, and dewpoint curves are indicated by T, Tf ,
and TD respectively. In figure 4-16, a marked warm front is approaching from the
south. Moderate continuous rain fell 2 hours later. At 1830 UTC, an aircraft
reported solid clouds from 1,000 to 44,000 feet (tropopause). The 1500 UTC
sounding shows an increasing dewpoint depression with height and no
discontinuity at the reported cloud top of 15,000 feet. A definite dry layer is
indicated between
Figure 4-17.-Example of inferring clouds from a RAOB with a
middle layer and no precipitation reaching the surface |
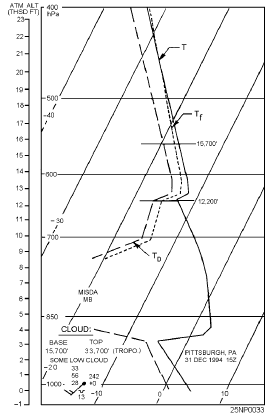 |
Figure 4-18.-Example of lnferring clouds from a RAOB showing
layer clouds with their Intermediate clear layers not showing in the
humidity trace. |
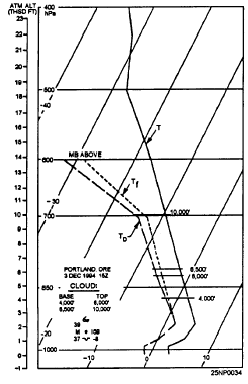 |
18,300 and 20,000 feet. The second reported cloud layer is indicated by a
decrease in dewpoint depression, but the humidity element is obviously slow in
responding. The dewpoint depression at the base of the cloud at 21,000 feet is
14°C and at 400-hPa; after about a 3-minute climb through the cloud, it is
still 10°C. From the sounding, clouds should have been inferred to be from
about 4,500 feet (base of the rapid humidity increase) to 500-hPa and a second
layer from 20,000 feet up. In view of the rapid falling of the cloud free gap
between 15,000 and 21,000 feet that followed as the warm front approached, the
agreement between reported and inferred conditions is good. Figure 4-17 shows a
middle cloud layer with no precipitation reaching the surface. This is a case of
a cloud in the 500-hPa surface with no precipitation reaching the surface; the
nearest rain reaching the surface was in Tennessee. The evidence from the
sounding for placing the cloud base at 12,200 feet is strong, yet the base is
inexplicably reported at 15,700 feet. The reported cloud base of 15,000 feet was
probably not representative, since altostratus, with bases 11,000 to 14,000 feet,
was reported for most stations over Ohio and West Virginia.
Figure 4-18 shows layered clouds with their intermediate clear layers not
showing in the humidity trace. There is good agreement between the sounding and
the aircraft report. The clear layer between 6,000 and 6,500 feet is not
indicated on the sounding. Thin, clear
layers, as well as thin cloud layers, usually cannot be recognized on the
humidity trace.
Comparisons between soundings and cloud reports provide us with the
following rules:
-
A cloud base is almost always found in a layer, indicated by the
sounding, where the dewpoint depression decreases.
-
You should not always associate a cloud with a layer of decreasing
dewpoint, but only when the decrease leads to minimum dewpoint
depressions from 6°C to 0°C. However, at temperatures below -25°C,
dewpoint depressions in clouds are often higher than 6°C.
-
The dewpoint depression in a cloud is, on the average, smaller in
clouds that have higher temperatures. typical dewpoint depressions are
1°C to 2°C at temperatures of 0°C and above, and 4°C between
-10°C and -20°C.
-
The base of a cloud
should be located at the base of the layer of decreasing dewpoint
depression, if the decrease is sharp.
-
If a layer of decreasing dewpoint depression is followed by a
layer of a stronger decrease, the cloud base should be associated with
the base of the strongest decrease
-
The top of a cloud layer
is usually indicated by an increase in dewpoint depression. Once a
cloud base is determined, the cloud is extended up to a level where a
significant increase in dewpoint depression starts. The gradual
increase of dewpoint depression with height in a cloud is not
significant.
|
In addition to the above analysis, another study was made to determine how
reliable the dewpoint depression is as an indicator of clouds. The results are
summarized in figure 4-19. Each graph shows the percent probability of the
existence of a cloud layer in January for different values of dewpoint
depression. On each graph one curve shows the probability of clear or scattered
conditions as a function of the dewpoint depression; the other curve shows that
of broken or overcast conditions.
Separate graphs are based on 1,027 observations, which are enough to
indicate the order of magnitude of the dewpoint depressions at the base of
winter cloud layers. Minor irregularities in the curves were not smoothed out
because it is not certain that they are all due to insufficient data. The graphs
are applicable without reference to the synoptic situation.
For a given winter sounding, you can estimate from the graph the probability
of different sky cover conditions with cloud bases between 1,000-hPa and 600-hPa
for layers of given minimum dewpoint depressions.
Figure 4-19.-Percent probability of existence of cloud layer
bases for different values of dewpoint depression (degrees C). Solid lines
represent probability of clear or scattered conditions; dashed lines, the
probability of broken or overcast conditions with the cloud layer bases
between 1,000-hPa and 600-hPa.
|
Studies of the humidity field
throughout frontal zones indicate there is a tongue of dry air extending
downward in the vicinity of the front, and sloping in the same direction as the
front. One study found that such a dry tongue was more or less well
developed for all frontal zones investigated. This dry tongue was best developed
near warm fronts; it extended, on the average, down to 700-hPa in cold fronts
and to 800-hPa in warm fronts. In about half the fronts, the driest air was
found within the frontal zone itself on occasion it was found on both the cold
and warm sides of the zone. About half the flights through this area showed a
sharp transition from moist to dry air, and the change in frost point on these
flights averaged about 20°C in 35 miles. Some flights gave changes of more than
20°C in 20 miles.
As a frontal cloud deck is approached, the dewpoint or frost point
depression starts diminishing rapidly. At distances beyond 10 to 15 nm, this
variation is much less. You should keep this fact in mind when attempting to
locate the edge of a cloud deck from rawinsonde data. Linear extrapolation or
interpolation of dewpoint depressions cannot be expected to yield good results.
For instance, when one station shows a dewpoint depression of 10°C and the
neighboring station shows saturation, the frontal cloud may be anywhere between
them, except within about 10 nm of the driest station.
Since the frontal cloud masses at midtropospheric levels is usually
surrounded by relatively dry air, it is possible to locate the edge of the cloud
mass from humidity data on constant pressure charts. This is so because the
typical change in dewpoint depression in going from the cloud edge into
cloudfree air is considerably greater than the average error in the reported
dewpoint depression.
Figure 4-20 shows an analysis of the 500-hPa dewpoint depression field
superimposed upon an analysis of areas of continuous precipitation, and of areas
of overcast middle clouds. The 500-hPa dewpoint depression isopleths were drawn
independently of the surface data. The analysis shows the following:
-
The regions of high humidity at the 500-hPa level coincide
well with the areas of middle clouds and the areas of precipitation.
-
The regions of high humidity at the 500-hPa level are separated from the
extensive dry regions by strong humidity gradients. These gradients are, in
all probability, much stronger than those shown on this analysis.
-
A dewpoint depression of 4°C or less is characteristic of the larger
areas of continuous precipitation, and also of the larger areas of overcast
middle clouds.
Figure 4-20.-Surface fronts, areas of continuous
precipitation, areas covered by 8/8 middle clouds, and isolines of 500-hPa
dewpoint depression at 0300 UTC, 7 September 1995
|
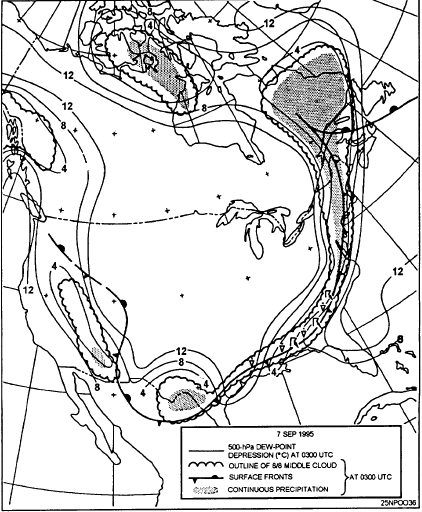 |
Since the 500-hPa level dewpoint depression analysis agrees well with the
surface analysis of middle cloud and precipitation, the possibility exists of
replacing or supplementing one of these analyses with the other. The
characteristics of the 500-hPa level dewpoint depression analysis, outlined
above, make it a valuable adjunct to the surface analysis. These analyses can be
compared and, by cross-checking, each can be completed with greater accuracy
than if they were done independently.
Using a single level (for example, the 500-hPa level dewpoint depression
analysis) to find probable cloud areas does not indicate clouds above or below
that level. For example, if the top of a cloud system reached only to 16,000
feet, and there was dry air above at 500-hPa (approximately 18,000 feet), you
wouldn't suspect, from the 500hPa analysis, the existence of clouds below the
500-hPa level.
However, an analysis of the extension of the moist layers in three
dimensions can be obtained simply by scrutinizing individual RAOBs. Those
selected should be in the general vicinity of, and the area 500 to 1,200 miles
upstream of, the area of interest, depending on the forecast period. The heights
of the bases and tops can be indicated, though there is little advantage in
indicating a dry layer 2,000 to 3,000 feet thick sandwiched between thicker
moist layers. Usually, it is sufficient to indicate the entire moist layer,
without bothering about any finer stratums. A survey of the cloud field is made
easier by writing the heights of the bases and tops in different colors.
A moist layer for the sake of simplicity may be defined as a layer having a
frost point depression of 3°C or less (i.e., a dewpoint depression of 4°C at
-10°C; 5°C at -20°C; 6°C at -30°C).
The type and intensity of precipitation observed at the surface is related
to the thickness of the cloud aloft, and particularly to the temperatures in the
upper portion of the cloud.
The results of a study relating
cloud-top temperatures to precipitation type and intensity are as follows:
-
From aircraft ascents through stratiform
clouds, along with simultaneous surface observations of precipitation,
it was found that 87 percent of the cases where drizzle
occurred, it fell from clouds whose cloud-top temperatures were warmer than
-5°C. The frequency of rain or snow increased markedly when the cloud-top
temperature fell below -12°C.
-
When continuous rain or snow
fell, the temperature of the coldest part of the cloud was below -12°C in
95 percent of the cases.
-
Intermittent rain was
mostly associated with cold cloud-top temperatures.
-
When intermittent rain
was reported at the surface, the cloud-top temperature was colder than
-12°C in 81 percent of the cases, and colder than -20°C in 63 percent of
the cases. From this, it appears that when minor snow (continuous or
intermittent) reaches the ground from stratiform clouds, the clouds (solid
or layered) extend in most cases to heights where the temperature is well
below -12°C, or even -20°C.
This rule cannot be reversed. When rain or snow is not observed at the
surface, middle clouds may well be present in regions where the temperature is
below -12°C or -20°C. Whether or not precipitation reaches the ground will
depend on the cloud thickness, height of the cloud base, and the dryness of the
air below the base.
Cirrus clouds form at temperatures of -40°C or colder. At these
temperatures, as soon as the air is brought to saturation, the condensate
immediately freezes. The ice crystals often descend in altitude slowly, to
levels that have air temperatures of -30°C, and persist if the humidity below
the formation level is high enough to support saturation. In general, cirrus
clouds are found in layers that are saturated, or supersaturated, with respect
to ice at temperatures colder than 0°C.
Cirrus, or cirriform clouds, are divided into three general groups: cirrus (proper),
cirrostratus, and cirrocumulus. Cirrus clouds, detached or patchy, usually do
not create a serious operational problem. Cirrostratus
and extensive cirrus haze, however, may be troublesome in high-level jet
operations, aerial photography, interception, rocket tracking, and guided
missile navigational systems. Therefore, a definite requirement for
cirrus cloud forecasting exists. The initial formation of cirrus clouds normally
requires that cooling take place to saturation, and to have temperatures near
-40°C. Under these conditions, water droplets are first formed, but most of
them immediately freeze. The resulting ice crystals persist as long as the
humidity remains near saturation with respect to ice. There is some evidence
that the speed of the cooling, and the kind and abundance of freezing nuclei,
may have an important effect on the form and occurrence of cirrus clouds. Slow
ascent starts crystallization at humidities substantially below saturation; this
is presumably the case in extensive cirrostratus clouds associated with warm
frontal altostratus clouds. If slow ascent occurs in air that has insufficient
freezing nuclei, a widespread haze may result, which at -30° to -40°C is
predominantly composed of water droplets. In the case of more rapid cooling,
there is a tendency for the initial condensation to contain a higher proportion
of water droplets, which leads to a "mixed cloud' that will convert to ice
or snow in time. Presumably, dense cirrus, fine cirrus, cirrocumulus, and anvil
cirrus clouds are of this type. It is assumed that fine cirrus clouds (proper)
are formed in shallow layers that are undergoing rapid convection due to
advection of colder air at the top of the shear layer.
On the other hand fine cirrus and cirrostratus clouds are so often associated,
and cirrostratus clouds are so often reported by pilots as developing from the
merging of fine cirrus clouds, that there is a question whether the process of
formation in cirrus and cirrostratus clouds are essentially different.
Nevertheless, the prevailing crystal types in cirrus and cirrostratus clouds
seem to differ, though this may not be universal, or may merely represent
different stages in cirrus cloud evolution.
Horizontal visibilities within cirrostratus clouds are generally between 500
feet and 2 nm. Thin cirrus haze, invisible from the ground, often reduces the
visibility to 3 nm.
A rule of thumb for forecasting or estimating the visibility within thin
cirrus or other high cloud (temperatures below -30°C) follows:
Visibility = 1/2 nm times dewpoint depression in degrees
C.
For example; temperature is -35°C, dewpoint is -38°C, and visibility = 1/2
x 3 = 1 1/2 nm.
This rule has been used successfully only in the Arctic where poor
visibility in apparently cloudfree air is often encountered.
Many forecasters have attempted to forecast cirrus clouds by using frontal
or cyclone models. This procedure is not always satisfactory. There are a number
of parameters, both surface and aloft, that have been correlated with cirrus
cloud formation. A few of the more prominent parameters are mentioned in the
following text.
Frontal and cyclone models have been developed that embody an idealized
cloud distribution. In these models, the cirrus clouds are lowering and
thickening to form altostratus clouds, which indicates an advancing warm
front.
Above 500-hPa the concepts of air
masses and fronts have little application. Most of the fine cirrus
clouds observed ahead of and above warm fronts or lows initially form
independent of the frontal middle cloud shield, though later it may trail
downward to join the altocumulus and altostratus cloud shields. With
precipitation occurring in advance of a warm front, a 60-percent probability
exists that cirrus clouds are occurring above. Cirrus clouds observed with the
cold front cloud shield either originate from cumulonimbus along and behind
the front or from convergence in the vicinity of the upper trough. In many
cases there is no post cold front cirrus clouds, probably due to marked
subsidence aloft.
One forecasting rule used widely
states that "the ridge line at 20,000 feet, about 500 hpa, preceding a
warm front marks the forward edge of the cirrus cloud shield."
For a typical 500-hPa wave pattern, the following information applies:
-
No extensive cirrostratus clouds will occur before the surface ridge
line arrives.
-
Extensive cirrostratus clouds follow the passage of the surface ridge
line.
-
No middle clouds appear before the arrival of the 500-hPa ridge line.
-
Middle clouds tend to obscure the cirrus clouds after passage of the
500-hPa ridge line.
-
When the 500-hPa wave has a small amplitude, the cirrus cloud arrival
is delayed and the clouds are thinner.
-
The greater the 500-hPa convergence from trough to ridge, the more
cirrus clouds between the surface and 500-hPa ridge lines.
Experiences of pilots have confirmed that the
tops of most cirrus clouds are at or below the tropopause. In the
midlatitudes, the tops of most cirrus cloud layers are at or within several
thousand feet of the polar tropopause. Patchy cirrus clouds are found between
the polar tropopause and the tropical tropopause. A small percentage of cirrus
clouds, and sometimes extensive cirrostratus, may be observed in the lower
stratosphere above the polar tropopause, but mainly below the level of the
jetstream core. The cirrus clouds of the equatorial zone also generally extend
to the tropopause. There is a general tendency for the mean height of the
bases to increase from high to low latitudes more or less paralleling the mean
tropopause height, ranging from 24,000 feet at 70° to 80° latitude to 35,000
to 4,000 feet or higher in the vicinity of the equator. The thickness of
individual cirrus cloud layers are generally about 800 feet in the
midlatitudes. The mean thickness of cirrus clouds tends to increase from high
to low latitudes. In polar continental regions in winter, cirrus clouds are
virtually based at the surface. In the midlatitudes and in the tropics, there
is little seasonal variation.
A discussion of cloud types associated with the jetstream is contained in
the AG2 TRAMAN, volume 1. In addition to this information, we will discuss a
few studies pertaining to cloud types. All of these studies agree that most
of the more extensive and dense clouds are on the equatorward of the jet axis.
The observed frequency of high clouds poleward of the jet axis can be
accounted for as the upper reaches of a cold front, or cold lows, not directly
related to the jetstream.
In some parts of a trough, these high clouds may tend to be dense, and in
other areas thin.
|